Resistive Random-Access Memory (ReRAM) is emerging as one of the most promising candidates for next-generation non-volatile memory technologies. The core principle behind ReRAM is its ability to change the resistance of a material by applying a voltage, a process known as resistive switching. This makes it ideal for memory applications as it combines high speed, low power consumption, and scalability. In this blog, we’ll take a deep dive into the resistive switching mechanism in ReRAM, exploring its working principles, materials, and underlying mechanisms that enable this technology to function.
What is Resistive Switching in ReRAM?
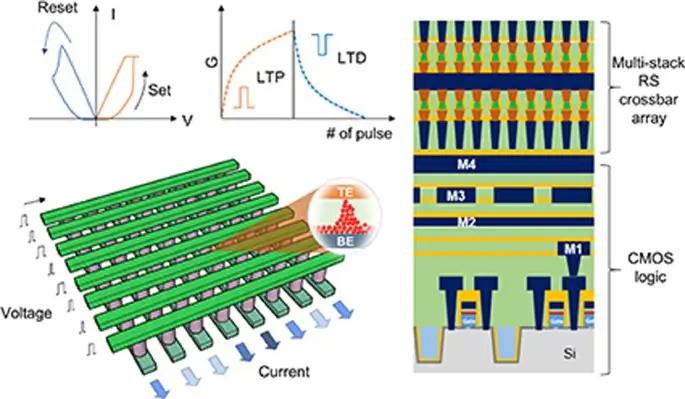
Resistive switching refers to the ability of a material to undergo reversible changes in resistance when an external voltage is applied. These changes in resistance can be classified into two states: a high resistance state (HRS) and a low resistance state (LRS). By controlling the voltage across the material, ReRAM devices can switch between these two resistance states, which corresponds to the process of storing and retrieving data.
In ReRAM, this resistive switching process typically occurs in metal-insulator-metal (MIM) structures, where the insulating material in between the two metal electrodes plays a crucial role in the switching behaviour.
Key Components of ReRAM
- Electrodes: These are the conductive layers, typically made from metals like platinum (Pt), silver (Ag), or tungsten (W). These electrodes are essential for applying the external voltage to the device.
- Dielectric/Insulating Layer: The insulating layer, often made of materials like titanium dioxide (TiO2), hafnium oxide (HfO2), or other transition metal oxides, is where the resistive switching takes place. The composition of this layer influences the switching performance.
- Voltage Source: An external voltage is applied across the MIM structure. Depending on the polarity and magnitude of the applied voltage, the resistance of the insulating layer can change, enabling data storage.
Resistive Switching Mechanism: The Basic Process
At the heart of ReRAM is the mechanism that governs the resistive switching. There are primarily two types of switching mechanisms:
1. Unipolar Switching
In unipolar switching, the resistance of the device is controlled by a voltage pulse of fixed polarity, but the magnitude of the voltage determines the switching behaviour. A voltage is applied to the MIM structure, and depending on the magnitude, it either lowers or raises the resistance of the dielectric layer. The switching occurs through the drift and migration of oxygen vacancies or metal filaments within the dielectric.
Process of Unipolar Switching:
- Set Process (High to Low Resistance): When a positive voltage of sufficient magnitude is applied, it results in the formation of conductive filaments (such as oxygen vacancies or metallic filaments) within the dielectric layer, which lowers its resistance, leading the device into the low resistance state (LRS).
- Reset Process (Low to High Resistance): When the polarity is reversed, a negative voltage is applied. This voltage causes the conductive filament to dissolve or disintegrate, raising the resistance back to its initial high resistance state (HRS).
2. Bipolar Switching
In bipolar switching, both the magnitude and polarity of the applied voltage are important in controlling the resistive switching. Unlike unipolar switching, where a fixed polarity is applied, in bipolar switching, positive and negative voltages are used to switch between the high and low resistance states.
Process of Bipolar Switching:
- Set Process: A positive voltage is applied across the device, which leads to the formation of conducting filaments within the dielectric, causing the device to transition from the HRS to the LRS.
- Reset Process: A negative voltage is applied, causing the conductive filaments to dissolve, thus restoring the device to its high resistance state.
The Role of Oxygen Vacancies and Metal Filaments
The resistive switching mechanism in ReRAM largely depends on the movement of oxygen vacancies or the formation and dissolution of metallic filaments within the dielectric material. This is an area of intense research, and understanding the dynamics of these materials is crucial for improving ReRAM performance.
Oxygen Vacancies:
- Forming Oxygen Vacancies: In metal oxide-based ReRAM, such as TiO2, the resistance changes due to the migration of oxygen vacancies under the application of an electric field. A positive voltage (during the “Set” process) typically drives the oxygen vacancies toward the cathode, reducing the resistance in the device by creating conductive paths.
- Vacancy Migration: When the polarity is reversed, the vacancies tend to move back toward the anode, increasing the resistance and returning the device to its high resistance state.
- Effect of Oxygen Vacancy on Conductivity: The concentration and distribution of oxygen vacancies in the dielectric layer directly influence the resistance states of ReRAM. A higher concentration of vacancies leads to lower resistance, whereas fewer vacancies increase the resistance.
Metal Filaments:
- Formation of Metal Filaments: In some ReRAM devices, especially those that use materials like silver or copper, the resistive switching occurs through the formation and dissolution of metallic filaments. When a voltage is applied, metal cations from the electrode diffuse into the dielectric, forming conductive filaments.
- Filament Growth: The filament growth typically starts from the electrode and extends into the dielectric material. This growth lowers the resistance of the device by creating a conductive bridge.
- Resetting the Filament: A reverse voltage can cause the metallic filament to dissolve or break, effectively restoring the device to its high resistance state.
Factors Affecting Resistive Switching
Several factors can influence the performance and reliability of resistive switching in ReRAM:
- Material Properties:
- The dielectric material’s composition, defect density, and crystalline structure significantly affect the switching behaviour.
- Materials like TiO2, HfO2, and WOx are commonly used due to their ability to form oxygen vacancies or metallic filaments.
- Voltage Magnitude and Pulse Duration:
- The magnitude and duration of the applied voltage pulses determine the formation, dissolution, and stability of the conductive filaments or oxygen vacancies.
- Electrode Material:
- The choice of electrode material impacts the ion migration, filament formation, and overall switching performance. Platinum and silver electrodes are commonly used, but emerging materials like graphene are also being explored.
- Switching Speed:
- ReRAM devices are known for their high switching speed, which makes them suitable for applications in high-speed memory and storage systems.
- Endurance and Retention:
- ReRAM devices must maintain their resistance states over time (retention) and withstand repeated switching cycles (endurance). These factors depend on the stability of the filaments or vacancy structures.
Advantages of Resistive Switching in ReRAM
- Non-volatility: Data is retained even when the power is turned off.
- High Speed: ReRAM offers fast switching times, making it suitable for high-speed memory applications.
- Low Power Consumption: ReRAM devices consume less power compared to traditional memory devices, especially during switching.
- Scalability: The simple structure of ReRAM devices allows for easy miniaturization, enabling higher memory densities.
- Endurance and Reliability: ReRAM can withstand a large number of write cycles, making it durable for long-term applications.
Applications of ReRAM
ReRAM’s ability to store data in a non-volatile manner and its speed make it an attractive candidate for a wide variety of applications, including:
- Next-Generation Memory Devices: ReRAM can serve as an alternative to Flash memory and DRAM, providing faster and more energy-efficient storage solutions.
- Neuromorphic Computing: The resistive switching mechanism mimics synaptic behaviour, making ReRAM a key technology for neuromorphic computing, where devices can simulate brain-like functions.
- Data Storage: ReRAM can be used for both permanent storage and fast cache memory due to its high-speed switching and low power consumption.
Conclusion
The resistive switching mechanism in ReRAM is a fascinating and complex process that holds the key to future non-volatile memory technologies. The ability to modulate the resistance of a material using an applied voltage, coupled with the unique role of oxygen vacancies or metal filaments, makes ReRAM a versatile and promising candidate for future computing needs. As research in this field continues, advancements in materials, device design, and fabrication processes are expected to unlock even greater potential, paving the way for faster, more efficient, and scalable memory solutions.