In the ever-evolving world of data storage, Resistive Switching Memory (ReRAM) has emerged as a promising alternative to traditional memory devices such as NAND Flash and DRAM. Among the various materials used in the development of ReRAM, Titanium Dioxide (TiO₂) has gained significant attention due to its excellent resistive switching properties, scalability, and potential for low-cost fabrication. This blog post delves into the applications, mechanisms, challenges, and future prospects of TiO₂ as a resistive switching memory device.
What is Resistive Switching Memory (ReRAM)?
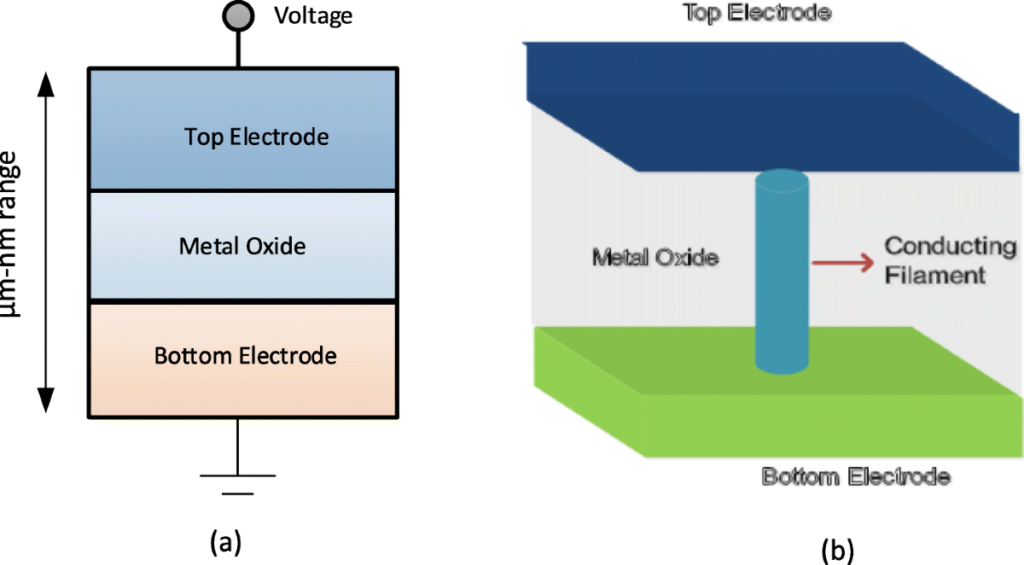
Resistive Switching Memory (ReRAM) is a type of non-volatile memory that relies on the ability of a material to change its resistance states when subjected to an external electrical field. These memory devices typically consist of a metal-insulator-metal (MIM) structure, where the insulator layer (the switching layer) undergoes resistance changes under the application of voltage. ReRAM is considered a potential successor to Flash memory because of its faster switching speeds, lower power consumption, and better scalability.
Working Principle of Resistive Switching
The resistive switching phenomenon involves the reversible change in the resistance of the switching layer, typically by forming and dissolving conductive filaments or by modulating the bulk properties of the material. Two primary modes of switching are observed:
- Unipolar Switching: In this mode, the device switches between two resistance states (high resistance state (HRS) and low resistance state (LRS)) based on the polarity of the applied voltage. The resistance change is irreversible with respect to the polarity of the voltage pulses.
- Bipolar Switching: In this mode, the resistance change occurs depending on both the magnitude and the polarity of the applied voltage. The switching is reversible, with the HRS and LRS being programmed by different voltage polarities.
TiO₂, as a transition metal oxide, exhibits distinct resistive switching behavior, which has made it a material of choice for ReRAM applications.
TiO₂ in Resistive Switching Devices
Titanium Dioxide (TiO₂) is an attractive material for ReRAM devices due to its tunable electronic properties, high dielectric constant, and stability. TiO₂ is a wide-bandgap semiconductor and can exist in multiple oxidation states, which makes it suitable for resistive switching applications. TiO₂ thin films can be easily synthesized using various deposition techniques, such as sputtering, pulsed laser deposition, atomic layer deposition (ALD), and chemical vapor deposition (CVD).
Key Properties of TiO₂ for ReRAM Applications
- High Switching Performance: TiO₂ has been shown to exhibit low operating voltages and high-speed switching, which are essential for memory devices. These properties result in faster data processing and lower power consumption, critical for advanced memory technologies.
- Multiple Oxidation States: TiO₂ exists in multiple oxidation states (Ti³⁺ and Ti⁴⁺), allowing it to undergo reversible redox reactions during the resistive switching process. This enables the creation of conductive filaments and the modulation of the material’s resistance.
- Scalability and Compatibility: TiO₂ is compatible with standard semiconductor fabrication processes and is scalable for integration into existing memory devices. Its high melting point and chemical stability also enhance its longevity and reliability.
- Non-Volatility: Like other memristive materials, TiO₂ retains its resistive state even after the power is switched off, making it ideal for non-volatile memory applications.
- Bi-Stable Resistive States: TiO₂ devices can exhibit bi-stable resistive states (HRS and LRS) that can be utilized to store binary data, a fundamental requirement for memory storage.
Mechanisms Behind Resistive Switching in TiO₂
The resistive switching in TiO₂ is primarily governed by two mechanisms: filamentary switching and interface-controlled switching. Understanding these mechanisms is key to optimizing TiO₂-based ReRAM devices.
1. Filamentary Switching
Filamentary switching involves the formation and dissolution of conductive filaments (usually oxygen vacancies) within the TiO₂ layer. The typical steps involved are:
- Voltage application: When a voltage is applied to the TiO₂ film, oxygen vacancies are created near the electrode interface (e.g., Ti electrode). This leads to a reduction of Ti⁴⁺ to Ti³⁺, creating a conductive filament.
- Forming process: In the forming process, a high voltage is applied to initiate the filament formation. Once the filament is established, the device switches to the LRS (low resistance state).
- Reset process: When the voltage polarity is reversed, the conductive filament is broken, and the device returns to the HRS (high resistance state).
- Set and Reset: The set process refers to the formation of the conductive filament, while the reset process involves the dissolution or annihilation of the filament, restoring the device to its high resistance state.
This filamentary mechanism is most commonly observed in TiO₂-based ReRAM devices and contributes to their high-speed switching and low power consumption.
2. Interface-Controlled Switching
In interface-controlled switching, the resistance change occurs due to the migration of oxygen vacancies at the TiO₂-electrode interface. Unlike filamentary switching, the resistance modulation in this case is not caused by the formation of a conductive filament but by changes in the distribution of oxygen vacancies near the interface. This type of switching is often observed in thin TiO₂ films, where the switching behavior can be controlled by adjusting the thickness of the dielectric layer.
Applications of TiO₂-Based ReRAM
1. Non-Volatile Memory (NVM)
TiO₂-based ReRAM devices are being actively explored as non-volatile memory (NVM) solutions due to their high density, fast switching speeds, and low power consumption. These devices can serve as a viable alternative to Flash memory, with potential advantages such as faster data transfer rates, better endurance, and higher scalability. Additionally, TiO₂-based ReRAM devices are already being integrated into multi-level cell (MLC) and 3D memory architectures for improved storage capacity.
2. Neuromorphic Computing
TiO₂-based ReRAM devices also show promise in neuromorphic computing, a field that mimics the behavior of the human brain for artificial intelligence (AI) applications. The resistive switching behavior of TiO₂ can emulate synaptic plasticity, which is the fundamental mechanism of learning in biological neural networks. By adjusting the resistance states of the TiO₂ memory elements, artificial neural networks can be trained for specific tasks, such as pattern recognition, image processing, and decision-making.
3. Embedded Systems and Edge Computing
With the increasing demand for edge computing devices, TiO₂-based ReRAM offers a potential solution due to its low power consumption and fast access times. These devices can be integrated into IoT (Internet of Things) applications, wearables, and smart sensors, where rapid data processing and non-volatile storage are crucial.
4. Data Storage and Logic Integration
TiO₂-based ReRAM devices can also be integrated into data storage and logic devices. Recent research has shown the possibility of combining memory and logic operations within a single device, paving the way for more efficient and compact computing systems. Such integration could be a key enabler for next-generation computing architectures, particularly in the realm of quantum computing and optoelectronics.
Challenges and Limitations of TiO₂-Based ReRAM
Despite its promising features, TiO₂-based ReRAM faces several challenges that need to be addressed for commercialization:
- Switching Stability and Variability: TiO₂-based ReRAM devices often suffer from switching instability and variability, which can affect the reliability and endurance of the memory devices. The formation of conductive filaments can be unpredictable, leading to performance degradation over time.
- Retention and Endurance: While TiO₂-based devices are non-volatile, their retention times (the ability to hold data over long periods) and endurance (the number of switching cycles) need to be further improved for practical applications.
- Scalability: As device dimensions shrink, the switching behavior can become more complex, and the electrical characteristics of the TiO₂ film may be influenced by factors such as film thickness and interface defects.
- Fabrication Complexity: The synthesis and fabrication of TiO₂-based ReRAM devices with precise control over thickness, uniformity, and material quality can be challenging and may require advanced deposition techniques, which could increase production costs.
Future Prospects
The future of TiO₂-based ReRAM devices looks promising, with ongoing research focused on improving switching performance, stability, and scalability. Advanced fabrication techniques, such as atomic layer deposition (ALD), could allow for precise control over the TiO₂ film’s thickness and composition. Researchers are also exploring hybrid structures, such as TiO₂ combined with other materials, to enhance the performance and functionality of ReRAM devices.
As we move towards increasingly complex and energy-efficient computing systems, TiO₂-based ReRAM could play a crucial role in the development of next-generation memory technologies, neuromorphic systems, and integrated memory-logic devices.
Conclusion
Titanium Dioxide (TiO₂) stands out as one of the most promising materials for resistive switching memory devices. Its excellent electrical properties, scalability, and non-volatile behavior make it an ideal candidate for a wide range of applications, including non-volatile memory, neuromorphic computing, and integrated logic-memory systems. While challenges such as switching stability and endurance remain, ongoing research and development efforts are likely to unlock the full potential of TiO₂-based ReRAM in the near future.