Supercapacitors, also known as ultracapacitors or electric double-layer capacitors (EDLCs), are a class of energy storage devices that have emerged as promising candidates for high-performance energy storage in various applications. Their unique ability to store large amounts of energy at high power densities and rapid charge/discharge cycles sets them apart from traditional batteries. As the world shifts towards renewable energy, electric vehicles, and other energy-efficient technologies, supercapacitors are becoming an increasingly important research field. This article provides an in-depth look at the current state of supercapacitor research, the materials and technologies that drive their performance, and the challenges and future directions for this rapidly advancing field.
Introduction to Supercapacitors
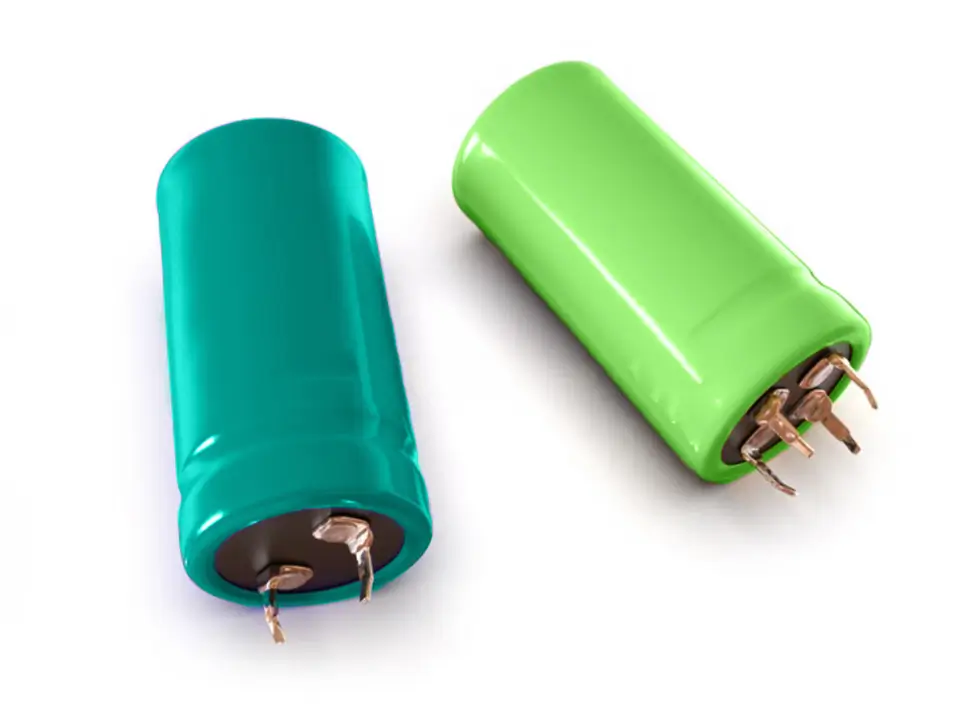
Supercapacitors store energy through electrostatic means, unlike conventional batteries, which rely on electrochemical reactions. They are characterized by their ability to charge and discharge much faster than batteries, making them ideal for applications requiring rapid bursts of energy. The fundamental principle behind a supercapacitor is the creation of an electric double layer at the interface between an electrode and an electrolyte, where energy is stored.
Supercapacitors are primarily categorized into two types based on their charge storage mechanism:
- Electric Double-Layer Capacitors (EDLCs): These capacitors store energy electrostatically by the formation of double layers of charge at the interface of the electrode and electrolyte. The energy storage is proportional to the surface area of the electrode and the voltage difference between the electrode and electrolyte.
- Pseudocapacitors: These capacitors store energy through a combination of electrostatic interactions and fast redox reactions at the surface of the electrodes. This gives them a higher energy density than traditional EDLCs, but they still offer relatively high power density.
Key Materials in Supercapacitor Research
The performance of supercapacitors depends largely on the materials used for their electrodes, electrolytes, and separators. Research into advanced materials plays a crucial role in improving the energy and power density, lifespan, and overall efficiency of supercapacitors.
1. Electrode Materials
The choice of electrode material is one of the most critical factors influencing the performance of a supercapacitor. The key characteristics of a good electrode material include a high surface area, good electrical conductivity, and the ability to undergo reversible charge storage processes.
a. Carbon-Based Materials
Carbon-based materials are the most commonly used for supercapacitor electrodes due to their high surface area, electrical conductivity, and stability. These include:
- Activated Carbon: The most widely used material in EDLCs, activated carbon is derived from organic precursors and has a large surface area, which is essential for energy storage.
- Graphene: Graphene, a single layer of carbon atoms arranged in a two-dimensional lattice, has attracted immense attention due to its exceptional electrical conductivity, large surface area, and mechanical strength. Researchers are exploring the use of graphene-based composites to improve the performance of supercapacitors.
- Carbon Nanotubes (CNTs): CNTs, with their high surface area and excellent conductivity, are another promising material for supercapacitor electrodes. They offer superior mechanical strength and flexibility compared to conventional carbon materials.
b. Transition Metal Oxides
Transition metal oxides, such as manganese oxide (MnO₂) and ruthenium oxide (RuO₂), are used in pseudocapacitors because of their ability to undergo fast redox reactions, which significantly increases the energy density compared to EDLCs. However, their high cost and limited cycling stability remain challenges that need to be addressed.
c. Conducting Polymers
Conducting polymers, such as polypyrrole (PPy), polyaniline (PANI), and poly(3,4-ethylenedioxythiophene) (PEDOT), are also used as electrode materials. These materials combine the high surface area and electrical conductivity of carbon with the pseudo-capacitive properties of redox reactions, making them an attractive choice for enhancing energy storage.
2. Electrolyte Materials
The electrolyte plays a critical role in determining the voltage window, stability, and overall performance of supercapacitors. Electrolytes are typically classified into three types:
- Aqueous Electrolytes: These are the most commonly used electrolytes in supercapacitors, offering high ionic conductivity and low cost. However, they are limited by a narrow voltage window (~1 V), which restricts the energy density of the supercapacitor.
- Organic Electrolytes: Organic electrolytes allow for a higher voltage window (typically up to 2.7 V), thus improving energy density. However, they have lower ionic conductivity compared to aqueous electrolytes.
- Ionic Liquids: Ionic liquids are a relatively new class of electrolytes that have gained attention due to their wide voltage window, high ionic conductivity, and thermal stability. They can be used in supercapacitors to increase energy density and improve performance under extreme conditions.
3. Separator Materials
The separator prevents direct contact between the two electrodes while allowing the movement of ions between them. Separators must be porous, chemically stable, and electrically insulating. Materials commonly used for separators include porous polymers, ceramics, and fibers.
Performance Metrics of Supercapacitors
Supercapacitors are evaluated based on several key performance metrics:
- Energy Density: Energy density is the amount of energy a supercapacitor can store per unit mass or volume. Supercapacitors typically have lower energy densities than batteries but can charge and discharge much more quickly.
- Power Density: Power density measures the amount of power a supercapacitor can deliver per unit mass or volume. Supercapacitors are known for their high power density, which makes them ideal for applications requiring rapid energy release.
- Cycle Life: Supercapacitors can typically undergo millions of charge-discharge cycles without significant degradation in performance, unlike batteries that experience a gradual decline in capacity over time. The long cycle life of supercapacitors makes them suitable for applications where frequent cycling is required.
- Voltage Window: The voltage window is the range of voltage within which a supercapacitor can operate safely without degradation. A wider voltage window results in higher energy density, and researchers are working to expand the voltage window of supercapacitors by improving electrolyte and electrode materials.
Current Challenges in Supercapacitor Research
Despite the significant progress made in supercapacitor technology, several challenges remain that researchers are working to overcome:
- Energy Density: While supercapacitors excel in power density, their energy density is still lower than that of batteries. To make supercapacitors viable for energy storage applications like electric vehicles (EVs), improving their energy density remains a key area of research.
- Cost: The use of expensive materials like ruthenium oxide and graphene in supercapacitors raises concerns about cost-effectiveness. The development of cheaper, scalable alternatives is essential for widespread commercialization.
- Stability and Longevity: Although supercapacitors have excellent cycle life, the stability of some materials, such as transition metal oxides, can degrade over time, especially under high power conditions. Improving the cycling stability of electrode materials is an ongoing challenge.
- Integration with Other Energy Systems: Integrating supercapacitors with other energy storage systems, such as batteries, to form hybrid systems is an area of significant interest. This could combine the high power density of supercapacitors with the high energy density of batteries to create more efficient energy storage solutions.
Future Directions in Supercapacitor Research
As the demand for energy storage solutions continues to grow, supercapacitors are expected to play an increasingly important role. Future research directions in supercapacitors include:
- Hybrid Supercapacitors: Combining supercapacitors with other energy storage devices, such as lithium-ion batteries or sodium-ion batteries, to create hybrid systems that offer both high energy density and high power density.
- Advanced Nanomaterials: Researchers are exploring the use of advanced nanomaterials, such as graphene-based composites, carbon nanofibers, and two-dimensional materials (e.g., MXenes), to improve the performance of supercapacitors.
- Flexible and Wearable Supercapacitors: The development of flexible supercapacitors that can be incorporated into wearable electronics and flexible devices is an exciting area of research. These devices could provide portable and efficient energy storage for a wide range of applications.
- Sustainability and Green Materials: There is increasing emphasis on developing environmentally friendly and sustainable materials for supercapacitors. This includes the use of bio-based materials, as well as recyclable and abundant resources, to reduce the environmental impact of supercapacitor production.
Conclusion
Supercapacitors are an exciting and rapidly advancing field of research that promises to revolutionize energy storage technology. While they are not yet a direct replacement for batteries in all applications, their unique combination of high power density, long cycle life, and fast charge/discharge capabilities make them indispensable for many modern technologies. As research continues to focus on improving energy density, reducing costs, and enhancing material performance, supercapacitors are poised to play a critical role in shaping the future of energy storage systems for applications ranging from portable electronics to electric vehicles and grid storage.