In recent years, the study of resistive switching materials has gained significant attention due to their potential applications in next-generation non-volatile memory devices, neuromorphic computing, and sensors. Titanium dioxide (TiO2) is one of the most promising materials for resistive switching (RS) applications. Its unique properties, such as high dielectric constant, ease of fabrication, and tunable resistive states, make TiO2 a standout candidate for these technologies. This blog aims to provide a comprehensive overview of TiO2 as a research material in the field of resistive switching applications, exploring its mechanisms, fabrication methods, challenges, and future potential.
What is Resistive Switching?
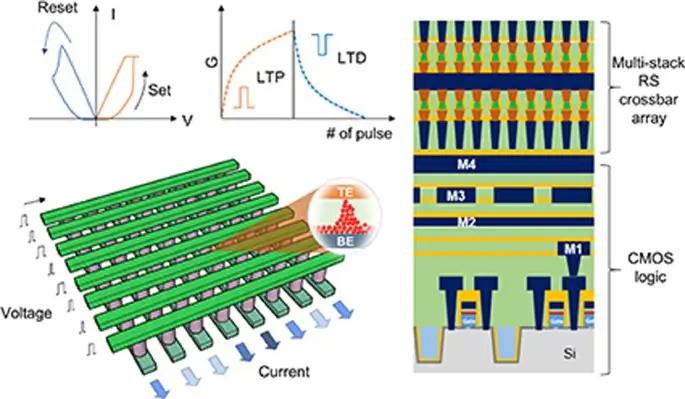
Resistive switching refers to the reversible change in the resistance of a material when an external voltage is applied. This phenomenon is central to the operation of resistive random-access memory (ReRAM) devices. Resistive switching typically occurs in two main modes:
- Unipolar switching: The resistance of the material changes when the applied voltage crosses a threshold in one direction, without requiring the voltage polarity to reverse.
- Bipolar switching: The material’s resistance changes when the voltage is applied in both positive and negative polarities, with the resistance switching occurring at both positive and negative threshold voltages.
The key to utilizing resistive switching in memory applications is the ability to induce and erase resistance states, which can represent binary data (0s and 1s).
Why TiO2?
Titanium dioxide (TiO2) has emerged as one of the leading materials for resistive switching due to its excellent electrical, optical, and mechanical properties. Some of the key attributes that make TiO2 an ideal candidate for resistive switching applications are:
- High Dielectric Constant: TiO2 has a high dielectric constant, which improves the retention and stability of the resistance states in memory devices.
- High Resistivity and Insulating Nature: As an insulator, TiO2 can be switched between two distinct resistive states (high resistance state and low resistance state), making it an excellent candidate for non-volatile memory applications.
- Nanostructure Compatibility: TiO2 can be easily fabricated into nanostructured thin films, which are crucial for miniaturizing memory devices, increasing their performance, and reducing power consumption.
- Oxidation States and Redox Activity: TiO2 exhibits different oxidation states of titanium (Ti^4+ and Ti^3+), which play a pivotal role in the resistive switching behaviour by enabling the migration of oxygen vacancies and the modulation of electronic conductivity.
- Thermal Stability: TiO2 is stable at high temperatures, making it suitable for high-performance applications, especially in harsh environments.
Mechanism of Resistive Switching in TiO2
The resistive switching behaviour in TiO2 is mainly driven by the migration of oxygen vacancies, which leads to a change in the material’s conductive properties. The general mechanism can be divided into two main processes: formation and rupture of conductive filaments.
1. Oxygen Vacancy Migration:
At the interface of TiO2 and the electrode, the applied electric field can induce the migration of oxygen vacancies. These vacancies are mobile due to the inherent defects in TiO2 and can move under an applied voltage, altering the local electronic properties of the material.
- In the high resistance state (HRS), the oxygen vacancies are dispersed, resulting in a relatively insulating state of TiO2.
- In the low resistance state (LRS), oxygen vacancies aggregate at the metal-TiO2 interface, creating conductive filaments that significantly reduce the resistance of the material.
2. Filamentary Model:
The resistive switching behaviour in TiO2 is often described using the filamentary model, which involves the formation and dissolution of conductive filaments made of oxygen vacancies. These filaments provide conduction paths, leading to a decrease in resistance in the ON state (LRS). The dissolution of these filaments returns the material to its OFF state (HRS).
The switching process typically requires two distinct steps:
- Set Process: A voltage of sufficient magnitude is applied to induce the migration of oxygen vacancies, forming a conductive filament and lowering the resistance of the TiO2 layer.
- Reset Process: A reverse voltage is applied, causing the dissolution of the conductive filament by driving the oxygen vacancies back, restoring the high resistance state.
Fabrication of TiO2-Based Resistive Switching Devices
The fabrication of TiO2-based resistive switching devices involves creating thin films of TiO2 on suitable substrates, followed by deposition of electrodes. These devices can be fabricated using a variety of techniques, including:
- Sputtering: A physical vapor deposition (PVD) technique commonly used for depositing thin TiO2 films onto substrates.
- Sol-Gel Process: A chemical method for fabricating thin films with high purity and uniformity. This technique is suitable for low-temperature applications.
- Atomic Layer Deposition (ALD): ALD allows for the precise control of film thickness at the atomic scale, making it ideal for fabricating uniform TiO2 layers for resistive switching applications.
- Electron-Beam Evaporation: Another method to deposit TiO2 thin films, which can be used to create high-quality films with controlled thickness.
- Nanostructured Films and Devices: TiO2 films can also be fabricated in nanostructured forms such as nanoparticles, nanowires, or nanotubes, which enhance the resistive switching properties by increasing surface area and reducing switching voltages.
Challenges and Limitations
While TiO2 holds great promise for resistive switching applications, several challenges need to be addressed for its widespread adoption in commercial devices:
- Cycle-to-Cycle Variability: TiO2-based devices often exhibit cycle-to-cycle variability in resistance switching, leading to issues with reliability and performance consistency. This is mainly due to the stochastic nature of oxygen vacancy migration and filament formation.
- Device Endurance and Retention: The long-term endurance (number of switching cycles) and retention (how long a resistance state is stable) of TiO2-based devices need to be improved. Repeated switching can lead to degradation of the TiO2 film or to the loss of oxygen vacancies, which affects performance.
- Control of Filamentary Growth: The formation of conductive filaments in TiO2 is not always uniform, and controlling their growth and dissolution is a challenge. Inhomogeneities in the distribution of oxygen vacancies can lead to non-ideal switching behaviour.
- Scaling Issues: As the demand for smaller and faster memory devices increases, the scaling of TiO2-based resistive switching devices presents challenges in terms of maintaining reliable performance at the nanoscale.
- Understanding of Switching Mechanisms: Although significant progress has been made in understanding the resistive switching mechanism in TiO2, the exact atomic-level processes and the role of various defects are still subjecting of ongoing research.
Future Directions and Potential Applications
The potential applications of TiO2-based resistive switching devices are vast and diverse. Some of the key areas where TiO2-based devices are expected to play a significant role include:
- Non-Volatile Memory (ReRAM): TiO2 is widely researched for use in resistive random-access memory (ReRAM) devices, which offer fast, low-power, and high-density memory storage alternatives to current memory technologies like Flash and DRAM.
- Neuromorphic Computing: The resistive switching behaviour of TiO2 can be harnessed to mimic synaptic behaviour in neuromorphic computing systems. TiO2-based devices can be used to create artificial synapses for brain-inspired computing, enabling more energy-efficient and adaptive computing systems.
- Sensors and Photodetectors: The tunable electrical properties of TiO2 can be exploited in gas sensors, humidity sensors, and photodetectors, where the resistive switching mechanism can be used to detect changes in environmental conditions.
- Memristors: TiO2-based memristors are a key component in the development of advanced computing architectures. Memristors, which are two-terminal devices that store information based on their resistance, can be used for memory storage, logic operations, and learning systems in next-generation computers.
- Energy-Efficient Electronics: Due to the low power consumption associated with resistive switching in TiO2, these devices are ideal candidates for energy-efficient electronics, including smart wearables, IoT devices, and low-power sensors.
Conclusion
Titanium dioxide (TiO2) stands out as a highly promising material for resistive switching applications, owing to its excellent electrical, optical, and structural properties. While challenges such as cycle-to-cycle variability, endurance, and device scaling remain, continued research and development efforts are likely to address these issues and lead to the commercialization of TiO2-based devices in memory, neuromorphic computing, and sensor applications. As we move toward a more connected and data-driven world, TiO2-based resistive switching devices could play a central role in shaping the next generation of electronic devices, providing high-speed, low-power, and non-volatile solutions for a range of applications.