RADAR, an acronym for Radio Detection and Ranging, is a technology that uses radio waves to detect and locate objects. It has a wide array of applications, from military and aviation to weather forecasting and speed enforcement. Understanding the physics behind RADAR involves delving into the principles of electromagnetic waves, signal processing, and the interaction of these waves with objects.
Basic Principles of RADAR
At its core, RADAR systems work by transmitting electromagnetic waves and analysing the waves that are reflected back from objects. The key principles involved include:
- Electromagnetic Waves: RADAR systems use radio waves, a form of electromagnetic radiation. These waves travel at the speed of light (approximately 3 x 108 meters per second) and can propagate through various media, including air, rain, and clouds.
- Transmission and Reception: A RADAR system consists of a transmitter, which emits radio waves, and a receiver, which detects the waves that are reflected back. The transmitter sends out pulses of radio waves in a specific direction. When these waves encounter an object, they are scattered in various directions. Some of the scattered waves are reflected back to the RADAR receiver.
- Time Delay and Distance Calculation: By measuring the time delay between the transmission of the pulse and the reception of the echo, the RADAR system can determine the distance to the object. This distance R can be calculated using the formula:
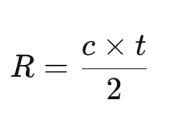
where 𝑐 is the speed of light and 𝑡 is the time delay.
4. Doppler Effect: RADAR can also determine the relative velocity of an object using the Doppler effect. When an object is moving, the frequency of the reflected waves changes. If the object is moving towards the RADAR, the frequency increases; if it is moving away, the frequency decreases. The change in frequency Δf is related to the velocity 𝑣 of the object by:
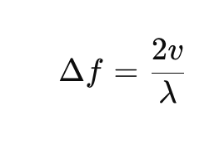
where 𝜆 is the wavelength of the transmitted waves.
Components of a RADAR System
A typical RADAR system includes several key components:
- Transmitter: Generates and amplifies the radio frequency (RF) signal. The transmitter can use various types of oscillators, such as magnetrons or klystrons, to produce the desired RF signal.
- Antenna: Radiates the transmitted RF signal into space and receives the reflected signals. Antennas can be parabolic dishes, phased arrays, or other configurations, depending on the application and required directivity.
- Receiver: Detects the RF signals reflected from objects and amplifies them for further processing. The receiver must be highly sensitive to detect weak echoes from distant objects.
- Duplexer: A switch that allows the same antenna to be used for both transmission and reception by alternating between the two modes.
- Signal Processor: Analyses the received signals to extract useful information, such as range, velocity, and angle of the detected objects. Modern RADAR systems use advanced digital signal processing techniques to enhance detection and reduce noise.
Types of RADAR Systems
- Continuous Wave (CW) RADAR: Emits a continuous signal and is used primarily for measuring velocity using the Doppler effect. It cannot measure distance directly.
- Pulsed RADAR: Emits short pulses of RF energy and measures the time delay of the reflected pulses to determine the distance to objects. This is the most common type of RADAR used in applications like air traffic control and weather monitoring.
- Frequency Modulated Continuous Wave (FMCW) RADAR: Combines aspects of both CW and pulsed RADAR by varying the frequency of the continuous wave. It can measure both distance and velocity and is commonly used in automotive and altimetry applications.
- Synthetic Aperture RADAR (SAR): Uses the motion of the RADAR antenna to simulate a large aperture and achieve high-resolution imaging. SAR is widely used in remote sensing and earth observation.
Interaction of Electromagnetic Waves with Objects
The behaviour of electromagnetic waves when they encounter objects is governed by several physical phenomena:
- Reflection: When an electromagnetic wave hits an object, part of the wave is reflected back towards the source. The strength of the reflection depends on the material properties and the surface roughness of the object.
- Refraction: The bending of waves as they pass through different media. This can affect the path of the waves and is particularly important in atmospheric RADAR applications.
- Diffraction: The bending of waves around obstacles and the spreading of waves when they pass through small openings. Diffraction can influence the detection capabilities of a RADAR system in complex environments.
- Scattering: The dispersion of waves in multiple directions due to irregularities on the surface of the object or within the medium. Scattering can provide information about the size and shape of the object.
Advanced RADAR Techniques
Modern RADAR systems employ various advanced techniques to enhance performance and accuracy:
- Pulse Compression: Involves modulating the transmitted pulse to achieve higher resolution. Techniques like chirp modulation can improve the ability to distinguish between closely spaced objects.
- Phased Array: Uses an array of antennas with variable phase shifts to electronically steer the beam without moving the antenna. This allows for rapid scanning and tracking of multiple targets.
- Adaptive Signal Processing: Involves dynamically adjusting the processing algorithms to optimise performance under changing conditions, such as varying levels of noise and clutter.
- Multiple Input Multiple Output (MIMO): Utilises multiple transmitters and receivers to improve spatial resolution and target detection capabilities.
Applications of RADAR
The versatility of RADAR technology leads to its use in a wide range of applications:
- Aviation: Air traffic control and collision avoidance systems rely on RADAR to ensure the safety of aircraft.
- Maritime: Ships use RADAR for navigation and collision avoidance, especially in poor visibility conditions.
- Meteorology: Weather RADAR systems detect precipitation, storm structures, and track severe weather phenomena like tornadoes and hurricanes.
- Military: RADAR is crucial for surveillance, target tracking, missile guidance, and stealth detection.
- Automotive: Advanced driver-assistance systems (ADAS) use RADAR for adaptive cruise control, collision avoidance, and autonomous driving.
- Space Exploration: RADAR is used for planetary exploration, satellite altimetry, and tracking space debris.
Conclusion
RADAR technology, grounded in the principles of electromagnetic wave propagation and reflection, is a powerful tool with diverse applications. From basic distance measurement to sophisticated imaging and velocity detection, RADAR systems have evolved to meet the needs of various industries. Understanding the physics behind RADAR not only illuminates its current capabilities but also paves the way for future innovations and advancements in this critical field.