Thermodynamics explores the transfer of heat energy between objects and the transformation of this energy into mechanical work, and vice versa.
Thermodynamics is a branch of physics that deals with the study of energy, its transformations, and the relationships between different forms of energy.
It plays a crucial role in understanding and predicting the behaviour of systems ranging from microscopic particles to large-scale industrial processes. Thermodynamics governs everything from the operation of engines to the behaviour of gases, liquids, and solids.
Basic Concepts
- Energy:
Energy is the capacity to do work or produce heat. It exists in various forms such as mechanical, thermal, chemical, electrical, and nuclear. Thermodynamics focuses on the conversion of energy from one form to another.
- System and Surroundings:
A system is a region of space under study, while the surroundings include everything outside the system that can interact with it. Systems can be classified based on their boundaries as open, closed, or isolated.
- State Variables:
State variables are properties of a system that describe its state. Examples include temperature, pressure, volume, and internal energy. These variables define the condition of the system at a particular moment.
- Processes:
A process is any change that occurs in a system. It can be characterized by the initial and final states of the system, as well as the path it follows. Processes can be classified as reversible or irreversible based on their reversibility.
Thermodynamic System:
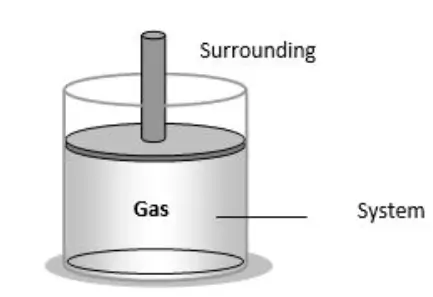
(i) A thermodynamic system comprises a vast assembly of atoms or molecules.
(ii) It is delimited by specific boundaries.
(iii) Surroundings refer to anything external to the thermodynamic system that exchanges energy or matter with it.
(iv) Thermodynamic systems come in three varieties:
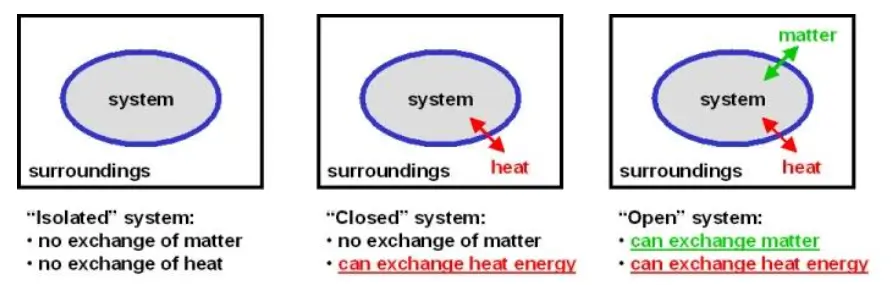
(a) Open System: These systems exchange both energy and matter with their surroundings.
(b) Closed System: These systems only exchange energy (not matter) with their surroundings.
(c) Isolated System: These systems do not exchange either energy or matter with their surroundings.
Thermodynamic Variables and Equation of State:
A thermodynamic system can be characterized by its pressure, volume, temperature, internal energy, and the number of moles. These parameters are termed as thermodynamic variables. The relationship between these variables (P, V, T) of the system is known as the equation of state.
For μ moles of an ideal gas, the equation of state is PV = μRT, and for 1 mole of an ideal gas, it is PV = RT.
Thermodynamic Equilibrium:
In a steady state, thermodynamic variables remain independent of time, and the system is considered to be in a state of thermodynamic equilibrium. For a system to be in thermodynamic equilibrium, the following conditions must be met:
(i) Mechanical equilibrium: No unbalanced forces exist between the system and its surroundings.
(ii) Thermal equilibrium: Uniform temperature prevails throughout the system, matching that of the surroundings.
(iii) Chemical equilibrium: Uniform chemical composition is maintained throughout the system and its surroundings.
Thermodynamic Process:
The alteration of a system’s state involves changes in thermodynamic variables such as pressure (P), volume (V), and temperature (T), known as a thermodynamic process. Some significant processes include:
(i) Isothermal process: Temperature remains constant.
(ii) Adiabatic process: No heat transfer occurs.
(iii) Isobaric process: Pressure remains constant.
(iv) Isochoric (isovolumetric process): Volume remains constant.
(v) Cyclic and non-cyclic process: In cyclic processes, initial and final states are identical, while in non-cyclic processes, they differ.
(vi) Reversible and irreversible process.
Indicator Diagram:
When the state of a gas (P, V, T) changes, it undergoes a thermodynamic process. The graphical representation of this change in state using pressure and volume is called an indicator diagram.
Zeroth Law of Thermodynamics:
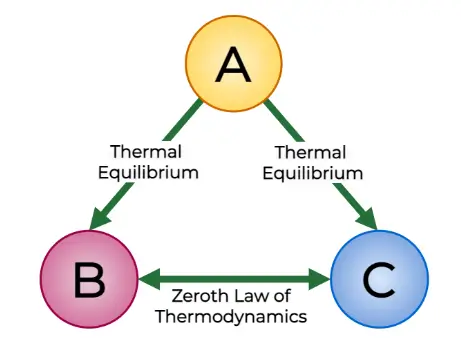
If systems A and B are each in thermal equilibrium with a third system C, then A and B are in thermal equilibrium with each other.
(1) The zeroth law gives rise to the idea of temperature. When all bodies are in thermal equilibrium, they share a common property with identical values. This property is known as temperature.
(2) Despite being discovered and numbered later than the first and second laws of thermodynamics, the zeroth law earns its name because it logically precedes them.
First Law of Thermodynamics (Law of Energy Conservation):
The total energy of an isolated system is constant. It can neither be created nor destroyed, only transformed from one form to another. This law is often expressed in terms of the conservation of internal energy and the concept of heat and work.
Second Law of Thermodynamics:
Heat flows spontaneously from a hotter body to a colder one, and not vice versa. It introduces the concept of entropy, which quantifies the disorder or randomness of a system. The second law also states that the entropy of a closed system tends to increase over time.
Third Law of Thermodynamics:
As the temperature of a system approaches absolute zero, the entropy of the system approaches a minimum value. This law provides an absolute reference point for entropy calculations and establishes the unattainability of absolute zero in finite steps.
Applications of Thermodynamics
- Heat Engines:
Thermodynamics principles are fundamental to the operation of heat engines, including steam engines, gas turbines, and internal combustion engines. These engines convert heat into mechanical work, enabling various forms of transportation and power generation.
- Refrigeration and Air Conditioning:
Thermodynamics principles govern the operation of refrigeration and air conditioning systems, which transfer heat from a cooler region to a warmer one. These systems find applications in food preservation, comfort cooling, and industrial processes.
- Chemical Reactions:
Understanding thermodynamics is essential for predicting the feasibility and spontaneity of chemical reactions. Concepts such as Gibbs free energy help determine whether a reaction will occur under given conditions and provide insights into reaction equilibrium.
- Material Science and Engineering:
Thermodynamics principles are crucial in material science and engineering for understanding phase transitions, alloy formation, and material properties. Thermodynamic models help optimise processes such as casting, welding, and heat treatment.
Conclusion
Thermodynamics provides a framework for understanding energy interactions and transformations in various systems. Its laws and principles have broad applications across science, engineering, and technology, shaping our understanding of the physical world and enabling the development of innovative technologies.
This overview should provide a solid understanding of the fundamentals of thermodynamics and its significance in various fields. Let me know if you’d like to dive deeper into any specific aspect!