Nuclear fusion, the process that powers the stars, holds immense promise as a sustainable energy source. Unlike nuclear fission, fusion combines atomic nuclei to release energy, producing minimal radioactive waste and virtually no greenhouse gases. One of the most critical aspects of achieving controlled fusion on Earth is plasma heating—raising the temperature of plasma to the millions of degrees required for fusion reactions. This blog delves into the science, techniques, and innovations in fusion plasma heating.
The Basics of Fusion and Plasma
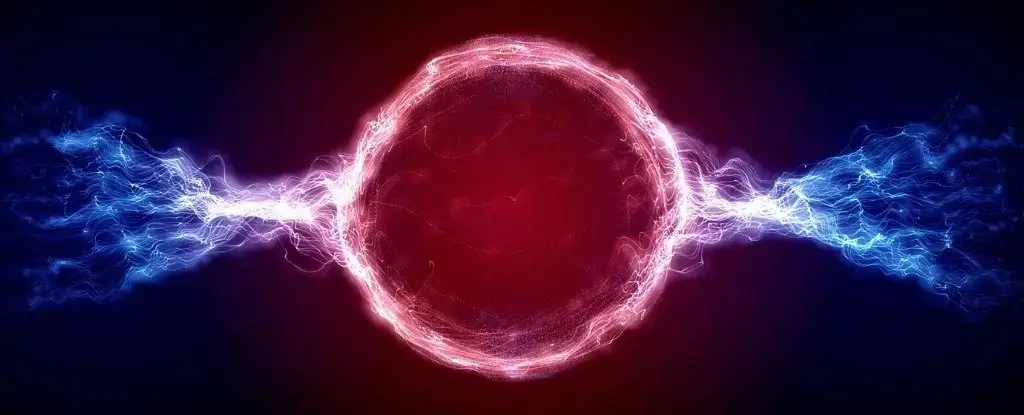
What is Nuclear Fusion?
Nuclear fusion involves fusing light nuclei, such as isotopes of hydrogen (deuterium and tritium), to form a heavier nucleus, releasing significant energy. For this to occur, the nuclei must overcome their electrostatic repulsion, requiring extremely high temperatures and pressures.
What is Plasma?
Plasma is the fourth state of matter, consisting of a hot, ionized gas of free electrons and ions. Achieving and sustaining plasma is a prerequisite for fusion since it allows nuclei to collide at high speeds, facilitating fusion reactions.
The Role of Plasma Heating
Fusion reactions require temperatures exceeding 100 million degrees Celsius—several times hotter than the sun’s core. Plasma heating methods are critical for achieving and maintaining these conditions in a controlled environment.
Techniques for Fusion Plasma Heating
1. Ohmic Heating
- Ohmic, or resistive, heating is one of the simplest methods for plasma heating. It involves passing an electric current through the plasma, generating heat due to resistance.
Limitations: This method becomes inefficient as plasma conductivity increases with temperature. At the high temperatures needed for fusion, additional heating methods are necessary.
2. Neutral Beam Injection (NBI)
- Neutral atoms are accelerated to high energies and injected into the plasma. Upon entering, these atoms collide with the plasma particles, transferring energy and heating the plasma.
Advantages: NBI provides both heating and additional plasma fueling.
Challenges: Designing systems that can accelerate particles to the required energy levels and efficiently neutralize the beams is complex.
3. Radiofrequency (RF) Heating
- Radio waves at specific frequencies are used to excite plasma particles, transferring energy and increasing the plasma temperature.
Types of RF Heating:
- Ion Cyclotron Resonance Heating (ICRH): Targets ions at their cyclotron frequency.
- Electron Cyclotron Resonance Heating (ECRH): Targets electrons at their cyclotron frequency.
Advantages: RF heating allows precise control of energy transfer to specific plasma particles.
4. Magnetic Confinement Heating
- Magnetic fields are used to confine and compress plasma in devices such as tokamaks and stellarators. Compressing the plasma increases its temperature.
Example: In tokamaks, magnetic fields also induce current for ohmic heating.
5. Microwave Heating
- High-power microwaves are directed into the plasma to excite particles, transferring energy effectively. This method is similar to RF heating but operates at higher frequencies.
6. Fusion Self-Heating (Alpha Heating)
- In advanced fusion scenarios, energy from the fusion reactions themselves (produced by fast-moving alpha particles) is used to sustain plasma temperatures.
Significance: Achieving self-heating is a milestone for creating a self-sustaining fusion reactor.
Innovations in Fusion Plasma Heating
High-Frequency Plasma Heating
Recent advancements in high-frequency heating techniques, such as advanced RF and microwave systems, have improved efficiency and control. High-frequency heating can target specific regions of plasma, optimizing the fusion process.
Auxiliary Heating Systems
Modern fusion reactors incorporate auxiliary systems that combine multiple heating methods. For example, ITER (International Thermonuclear Experimental Reactor) will use a combination of ohmic, RF, and neutral beam heating.
Advanced Plasma Diagnostics
Sophisticated diagnostics allow real-time monitoring of plasma conditions, enabling precise control of heating processes. These tools help researchers understand plasma behavior, improving heating efficiency and stability.
Laser-Induced Plasma Heating
High-power lasers are being investigated for plasma heating in inertial confinement fusion (ICF). This method uses intense laser pulses to compress and heat fuel pellets, achieving the extreme conditions necessary for fusion.
Plasma Heating in Fusion Devices
Tokamaks
Tokamaks are donut-shaped reactors that use magnetic fields to confine plasma. They rely on a combination of ohmic heating, NBI, and RF heating to reach fusion temperatures. ITER, the largest tokamak under construction, aims to demonstrate the feasibility of fusion power.
Stellarators
Stellarators use complex magnetic field geometries for plasma confinement. Although less reliant on plasma currents, stellarators benefit from similar heating techniques as tokamaks.
Inertial Confinement Fusion (ICF)
ICF involves compressing fuel pellets with lasers or particle beams. Plasma heating in ICF is achieved through direct energy transfer from the laser or beam to the fuel.
Challenges in Plasma Heating
1. Energy Efficiency
- Plasma heating methods consume significant energy. Achieving a net-positive energy output—where fusion generates more energy than is consumed—is a key challenge.
2. Plasma Instabilities
- Heating plasma can lead to instabilities, such as turbulence and disruptions, which hinder confinement and reduce heating efficiency.
3. Material Limitations
- The extreme temperatures required for fusion can damage reactor materials. Developing heat-resistant and durable materials is critical.
4. Scaling to Commercial Reactors
- Translating experimental plasma heating techniques into scalable solutions for commercial reactors remains a significant hurdle.
The Future of Plasma Heating
Artificial Intelligence in Plasma Control
AI and machine learning are being used to optimize plasma heating and confinement. Predictive models help manage instabilities and improve the efficiency of heating systems.
Advanced Superconductors
New superconducting materials are enabling stronger magnetic fields for better plasma confinement and heating in fusion devices.
Compact Fusion Devices
Innovations in plasma heating are driving the development of compact fusion reactors, such as those being pursued by companies like Tokamak Energy and Commonwealth Fusion Systems.
Why Plasma Heating Matters
Fusion plasma heating is not just a scientific endeavor; it is a crucial step toward solving global energy challenges. Fusion offers a virtually limitless, clean, and safe energy source, capable of meeting the growing demand without harming the environment. Innovations in plasma heating bring us closer to realizing this potential, making it a critical area of research and development.
Conclusion
Fusion plasma heating is at the heart of achieving controlled nuclear fusion. From traditional methods like ohmic heating to advanced techniques like laser-induced heating, each innovation brings us closer to unlocking the power of the stars. While challenges remain, the progress in plasma heating technology underscores the promise of fusion as the ultimate energy solution. With continued investment in research and collaboration among scientists worldwide, the dream of fusion energy is no longer a distant vision—it is within reach.