In the realm of atomic and molecular physics, one of the fundamental principles governing the behaviour of particles is the Pauli Exclusion Principle. This principle, formulated by the renowned physicist Wolfgang Pauli in 1925, plays a crucial role in understanding the electronic structure of atoms and the properties of matter. From the arrangement of electrons in atoms to the stability of matter, the Pauli Exclusion Principle underpins various phenomena in the microscopic world.
The Principle Explained:
The Pauli Exclusion Principle states that no two electrons in an atom can have the same set of quantum numbers. Quantum numbers are parameters that describe the quantum state of an electron, including its energy, angular momentum, magnetic moment, and spin. According to the principle, no two electrons within an atom can occupy the same quantum state simultaneously.
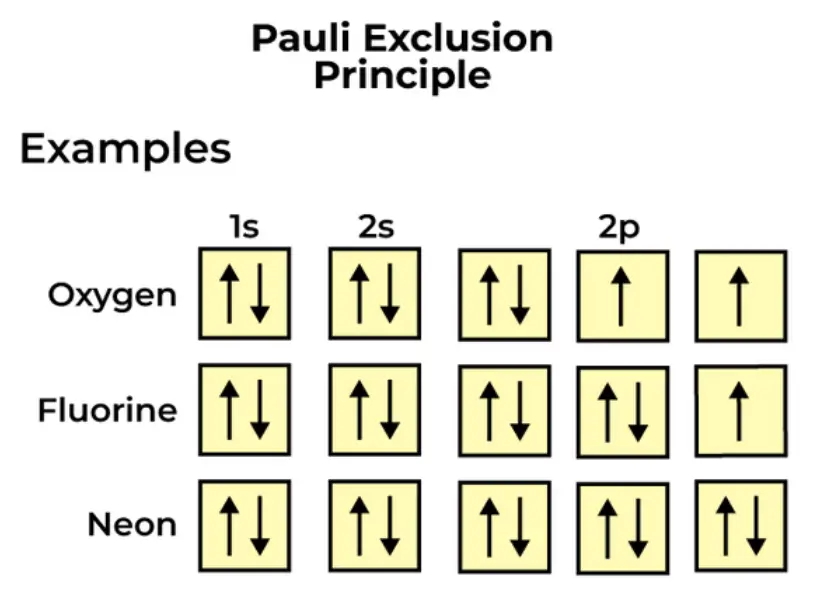
Pauli’s Exclusion Principle states that no two electrons in the same atom can have identical values for all four of their quantum numbers.
In other words,
- No more than two electrons can occupy the same orbital and
- Two electrons in the same orbital must have opposite spins.
Implications in Atomic Structure:
In the context of atomic structure, the Pauli Exclusion Principle determines the arrangement of electrons in atomic orbitals. Each orbital can accommodate a maximum of two electrons with opposite spins. This leads to the filling of electron shells and subshells in a specific order, known as the Aufbau principle. For instance, in the ground state of a carbon atom (atomic number 6), the two electrons in the 1s orbital have opposite spins, while the remaining four electrons fill the 2s and 2p orbitals according to their increasing energy levels and with opposite spins due to the Pauli Exclusion Principle.
Role in Chemical Bonding:
The Pauli Exclusion Principle also influences chemical bonding and the formation of molecules. When atoms combine to form molecules, their valence electrons participate in bonding. However, these electrons must obey the exclusion principle, preventing the overlap of electron states within the same atom. As a result, the formation of covalent bonds between atoms is governed by the availability of unoccupied molecular orbitals that satisfy the exclusion principle. For example, in the formation of a water molecule (H2O), each hydrogen atom shares its electron with the oxygen atom, resulting in a stable molecule obeying the Pauli Exclusion Principle.
Application in Fermionic Systems:
Beyond atomic and molecular physics, the Pauli Exclusion Principle has broader implications in understanding the behaviour of fermionic particles. Electrons, being fermions, follow this principle, but it also applies to other particles with half-integer spins, such as protons, neutrons, and quarks. This principle governs the behaviour of matter at low temperatures, such as in Fermi gases and degenerate matter, where the exclusion of quantum states leads to phenomena like electron degeneracy pressure in white dwarfs and neutron stars.
Example:
Consider the helium atom, which consists of two protons, two neutrons, and two electrons. The Pauli Exclusion Principle dictates that the two electrons in the helium atom must occupy different quantum states. Therefore, one electron occupies the 1s orbital with spin up, while the other electron occupies the same orbital with spin down. This arrangement satisfies the exclusion principle, ensuring the stability of the helium atom.
Conclusion:
The Pauli Exclusion Principle stands as a cornerstone in atomic and molecular physics, shaping our understanding of the behaviour of electrons and other fermionic particles. Its implications extend from the electronic structure of atoms to the stability of matter and the properties of exotic systems in astrophysics. By obeying this principle, particles adopt unique quantum states, leading to the diverse array of structures and phenomena observed in the microscopic world.